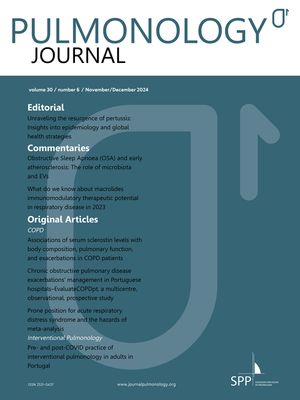
High generated tidal volumes (Vt) have been correlated with higher risk of self-induced lung injury and worse clinical outcome. This study aimed to evaluate the effectiveness and safety of a new helmet continuous positive airway pressure delivered (h-CPAP) configuration allowing Vt monitoring in patients affected by COVID-19.
MethodsThis prospective observational study was performed in the respiratory intermediate care unit of University Hospital in Turin, Italy, between March 24th, and June 15th, 2020. Included patients were treated with CPAP via a single-limb intentional leak configuration by a turbine-driven ventilator, provided with a dedicated patch. Effectiveness and safety of the configuration and healthcare workers safety were the outcomes of the study.
Main findingsThirty-five patients were included in this study. Median age was 67 years (IQR 57–76 years), and 30 patients (85.7%) were men. Median value of overall leaks (intentional plus unintentional) was 68 L/min (IQR 63–75). Reliability of Vt measurements was 100%. An out of scale of Vt (above 50% compared to the previous values) was never recorded. Six patients (17.1%) needed more than two helmet replacements, due to leak test >10 l/min. Arm oedema and skin breakdowns were reported in sixteen (45.7%) and seven (20%) patients respectively. Among the 63 healthcare workers involved in the care of COVID-19 patients during the study only one was positive at RT-PCR nasopharyngeal swab testing.
ConclusionsThe use of h-CPAP for treating COVID-19 in this configuration allowed for reliable Vt monitoring. Further studies evaluating this configuration in larger patients’ cohorts are needed.
As of March 2021, almost 117 million confirmed cases of coronavirus diseases (COVID-19) have been reported, including more than 2.5 million deaths.1 SARS-CoV-2 is responsible for acute respiratory failure (ARF) in nearly 20% of cases,2 often leading to endotracheal intubation and admission to intensive care units (ICUs).
Noninvasive respiratory supports (NRS), including noninvasive continuous positive airway pressure (nCPAP) have been proposed for the treatment of patients with COVID-19 related ARF.3–6 Respiratory Intermediate Care Units (RICUs) have been implemented to extensively offer NRS under the supervision of experienced teams with close monitoring.
The use of NRS5,7 may pose an additional risk of infection for healthcare workers. Helmet CPAP (h-CPAP)8–14 may reduce aerosolization and provide better comfort for patients,8–10,15 in comparison with face masks. In patients receiving NRS, high tidal volumes have been correlated with large diaphragmatic swings, higher risk of self-induced lung injury,16 and worse clinical outcomes.17 Thus, the measurement of tidal volume (Vt) may be clinically important to minimize the risk of self-induced lung injury and help clinicians recognize a patient's respiratory drive or respiratory compliance worsening. So far, h-CPAP did not allow measurement of Vt due to its intrinsic mechanical properties.18,19 Recently, a new configuration of h-CPAP delivered using a turbine driven ventilator with a single-limb intentional leak configuration has been described.18 This configuration, provided with dedicated software, has allowed for an accurate estimation of Vt, intentional and unintentional leaks.18 This study aimed to clinically evaluate the effectiveness and the safety of this h-CPAP configuration 18 allowing Vt monitoring in patients with COVID-19 related ARF in a RICU setting.
MethodsThe study was conducted after the approval of the Ethics Committee of the study center (CORACLE Registry – “Città della Salute e della Scienza di Torino” University Hospital, ID number 0031285, date 24 March 2020), in accordance with the Helsinki Declaration and written informed consent were collected from all patients or legal representatives. We adhered to the STROBE statement (Strengthening the reporting of observational studies in Epidemiology) (see the STROBE checklist in the Supplementary material A)
Study design, setting and patient cohortThis prospective single-centre observational study was performed in the RICU of “Città della Salute e della Scienza di Torino” University Hospital, Turin, Italy, between March 24th, and June 15th, 2020.
All the consecutive patients admitted to the study center were assessed for eligibility. Inclusion criteria were: age ≥ 18 year-old, clinical diagnosis of acute respiratory failure caused by SARS-CoV-2 (confirmed by RT-PCR on rhino-pharyngeal swab), PaO2:FiO2 ratio <250 mmHg during an oxygen supplementation trial of at least one hour with a Venturi mask (FiO2>40%) or non-rebreathing mask, with respiratory distress (respiratory rate (RR) >25 breaths/minute, moderate dyspnoea as measured by the BORG [20]) and evidence of bilateral infiltrates at chest X-ray and/or computed tomography (CT).
Patients were excluded if they met one or more of the following criteria: need for intermittent positive airway pressure ventilation (NIPPV) for hypercapnic respiratory failure (PaCO2>45 mmHg), a former trial of h-CPAP or helmet NIPPV > 24 h in the emergency department or in other settings before RICU admission.
Study intervention and co-interventionsIncluded patients were treated in CPAP mode via a single-limb intentional leak configuration, by a turbine-driven ventilator (Philips V60 or TROLOGY EVO Respironics Ventilator) provided with a dedicated patch. Detailed description of this configuration has been previously published.18,21 In this configuration, the helmet expiratory port was capped with a connector having a 6 mm internal diameter hole to provide the intentional leak as previously described18 (Fig. 1). A high oxygen pressure inlet allowed the ventilator to guarantee a pre-set stable FiO2. The ventilators’ monitoring system ensured continuous monitoring of the overall leaks (intentional and unintentional), inspired Vt estimation, pressure and flow curves tracings. Helmets (Dimar, medical Device, Medolla (MO) Italy or CaStar StarMed, Intersurgical, Mirandola, Italy) were secured by padded armpit braces and size was chosen according to patient's neck diameter. When available, helmets equipped with an inflatable neck cushion were used to limit the unintentional leak.12 The helmet ports were provided with High-Efficiency Particulate Air (HEPA) filters to limit aerosolization.
Patients included in the study were treated with continuous h-CPAP (24 h/24 h) for at least 48–72 h. When tolerated, prone positioning was kept for at least 2 h three times per day.22 Positive pressure was chosen between 8 and 15 cmH2O and FiO2 set at the lowest possible value to achieve oxygen saturation ≥96%.
Patients on h-CPAP who did not show signs of respiratory distress (e.g. RR <25 or use of accessory muscles) and were able to maintain a SpO2>94% with a FiO2<50% and a PEEP<10 cmH2O underwent a weaning trial in HFNT or Venturi mask. Criteria to consider a patient as successfully weaned from h-CPAP was ability to maintain a PaO2:FiO2 ratio >250 on Venturi mask with a FiO2 <40% for at least 24 h.
A “leak test” (<10 l/min threshold) was periodically performed by closing with a finger (for 5–10 s) the 6 mm hole of the expiratory port. As the ventilator measured the overall leaks, unintentional leaks coming from the helmet collar were evaluated when intentional leaks (coming from the expiratory port) were excluded after expiratory port closure. At the end of each test, overall leaks were recorded. The continuous monitoring of the overall leak (unintentional plus intentional) allowed the early identification of values out of the range (greater than 30% compared to previous values). In these cases, the leak test (occlusion of the intentional leak and check of unintentional leak on the monitor) helped confirm the possible displacement or damage of the helmet. Patients received sedation in case of Richmond Agitation Sedation Score (RASS) ≥ 1.23 A detailed description of healthcare workers protection policy in our centre is provided in Supplementary material B.
Study endpointsTreatment effectiveness was evaluated as:
- Reliability of Vt measurement as defined as an out of scale Vt (>50%) compared to the mean value recorded on the previous hours;
- Proportion of patients showing lack in improvement in arterial blood gases due the interface setup malfunctioning (i.e. unintentional leak leading to a lack to maintain a correct ventilatory support)24,25;
- Unintentional leak as measured by the leak test and dynamically by ventilator monitoring system needed helmet repositioning
Patient's treatment safety was evaluated as:
- Rate of adverse events due the helmet (i.e. skin breakdown)26–28
- Rate of suffocation or death due to the interface29
Healthcare workers safety was evaluated as:
- SARS-CoV-2 infection rate among RICU staff (symptomatic or asymptomatic infection confirmed by RT-PCR test on rhino-pharyngeal swab) during the study period.
Secondary endpoints of the study included:
- Improvement in arterial blood gases (PaO2, PaCO2, PaO2:FiO2);
- Need for sedation due the interface intolerance (to reach a target RASS 0/-1)
- Rate of failure (as defined as need to switch to a facial mask) due the interface intolerance
- Respiratory rate and dyspnoea (according to Borg scale);
- Rate of intubation not correlated to the interface setup (detailed criteria for intubation are described in Supplementary material B)
- Death during RICU stay (not correlated to the interface setup).
- Other adverse events not correlated to the interface setup (e.g. cardiovascular events, pulmonary embolism, acute kidney injury etc.)
Arterial blood gas analyses were recorded at admission before starting h-CPAP (T0), within 6 h (T6), and on day 1 (T24) after h-CPAP initiation. Vital parameters (systemic blood pressure - SBP, heart rate - HR, respiratory rate - RR, tidal volume – Vt) were monitored continuously and recorded together with the assessment of the dyspnoea score, as measured by the BORG20 scale, three times a day until the start of the weaning, then once a day.
Due to the nature of the study, a convenience sample of consecutive patients was included, and no a priori sample size calculation was done.
Continuous variables were expressed as median values with interquartile range (IQR), and categorical variables were expressed as proportions. Comparisons between groups were performed for continuous variables using the Wilcoxon rank-sum test. Variance analysis (ANOVA) test was used to compare results of repeated measures (T0, T6, T24). Categorical variables were examined using the Fisher's exact test. All statistical analyses were performed using STATA 13.1 (StataCorp, College Station, Tx, USA).
ResultsBaseline characteristics of study cohortSeventy-one patients were admitted to the study centre during the period of study. A total of thirty-five patients (49%) were eligible and all were included in this study.
Baseline characteristics and blood gas analysis of the included patients at admission are showed in Table 1. The median age was 67 years (IQR 57–76), and 30 patients (85.7%) were men. At admission, patients showed a median PaO2/FiO2 129 (IQR 89–166), signs of respiratory distress (RR median 32, IQR 28–35) with moderate dyspnoea (BORG median 3, IQR 2–4). Details on the co-treatments received by the included patients are provided in the Supplementary material C, Table C.1.
Presenting characteristics of patients with COVID-19 at admission in RICU (T0).
APACHE II Acute Physiology and Chronic Health Evaluation II score, BMI Body mass index, BORG Modified Borg Dyspnea Scale (MBS), COPD Chronic obstructive pulmonary disease, CPAP Continuous Positive Airway Pressure, CRP C-reactive protein, HCO3- bicarbonate, FiO2 fractional inspired oxygen, PaCO2 arterial partial pressure of carbon dioxide, PaO2 arterial partial pressure of oxygen, RICU respiratory intermediate care unit, RR respiratory rate, SAPS II Simplified Acute Physiology score II, SOFA Sequential Organ Failure Assessment score
Table 2 summarizes the results for primary and secondary outcomes. Reliability of Vt measurements was 100%. An out of scale of Vt (above 50% compared to the previous values) was never recorded in any patient at any time-point. Fig. C.1 (Supplementary material C) shows the trend of the recorded Vt of each patient during continuously hCPAP and weaning. No patients showed a lack of arterial blood gases improvement due to the configuration set up, with overall leaks reaching the safety threshold value of the turbine driven ventilator. Median value of overall leaks (intentional plus unintentional) was 68 L/min (IQR 63–75). Two patients (5.7%) reported intolerance to the helmet, and they alternated it with the mask without CPAP interruption.
Primary and secondary endpoints results.
ABG arterial blood gases, DNI do-not-intubate decision, EPUAP European Pressure Ulcer Advisory Panel (1), HCW Health care workers, HFNT High Flow Nasal Therapy, ICU Intensive care unit, NC standard nasal cannula, RICU Respiratory intermediate care unit, VM Venturi mask.
No cases of suffocation or death due to the interface occurred. Six patients (17.1%) needed more than two helmet replacements every three hours, due to leak test >10 l/min. Arm oedema and skin breakdowns were reported in sixteen (45.7%) and seven (20%) patients respectively.
Among the 63 healthcare workers involved in the care of COVID-19 patients during the study period, 3 workers (4.8%) showed positive SARS-CoV-2 IgG. Of these, only one was positive at RT-PCR nasopharyngeal swab testing. All the workers remained asymptomatic during the study period. The study was conducted during the first wave of pandemic in the north of Italy, when the pandemic was out of control. Thus, it cannot be defined if the infection occurred inside or outside the hospital.
Thirteen patients (37.1%) required sedation with morphine and/or dexmedetomidine to improve the tolerability of continuous h-CPAP and reach the target of RASS 0/-1. Adverse events not related to the helmet configuration are reported in Table C.2, Supplementary material C. Table 3 shows respiratory parameters at admission in RICU (T0), after 6 h (T6), and after 24 h (T24) since the start of helmet CPAP. Respiratory parameters at the start and at the end of helmet CPAP weaning are shown in Table C.3, Supplementary material C. Table C.4 and Table C.5 (Supplementary material C) respectively show the patients who died in RICU (DNI) (n = 3) and the respiratory parameters of intubated patients (n = 9). Both patients successfully completing (S) and failing (F) h-CPAP showed an improvement of PaO2/FiO2 ratio, respiratory rate and dyspnoea within the first 6 and 24 h of treatment initiation. PaCO2 increased significantly after 6 h only in patients successfully completing h-CPAP, and in both groups after 24 h. The only difference observed between success and failure was PaO2/FiO2 ratio at 24 h (S: 257 (IQR 181–319) vs F: 171 (IQR 133–225), P = 0.02) (Table C.6, Supplementary material C). Vt, reported as ml/IB, was not significantly different at 6 and 14 h between patients who completed or failed h-CPAP (Table C.6, Supplementary material C).
Secondary endpoints results - Respiratory parameters at admission in RICU (T0), after 6 hours (T6), and after 24 h (T24) since the start of helmet CPAP.
* Variance analysis (ANOVA) for repeated measures (T0, T6, T24).
# Wilcoxon Test signed rank test for paired measures.
PaCO2 arterial partial pressure of carbon dioxide, PaO2 arterial partial pressure of oxygen, FiO2 fractional inspired oxygen, HCO3− bicarbonate, RR respiratory rate, BORG Modified Borg Dyspnea Scale (MBS), Vt Tidal volume, Ve minute volume IBW Ideal Body Weight.
Our data showed the effectiveness and safety of h-CPAP for patients with COVID-19 related ARF in a RICU setting, using a high-performance turbine-driven ventilator in a single-limb intentional leak new configuration allowing Vt monitoring. This is the first clinical study evaluating this new h-CPAP configuration.18
The measurement of Vt was accurate throughout the study period, and no major flaws concerning unintentional leak affected estimates. Accurate Vt monitoring is clinically important to minimize the risk of Self-Inflicted Lung Injury (SILI) avoiding the generation of high Vt, while limiting unintentional leaks. The helmet may provide better tolerability and fewer unintentional leaks11,30 than mask interfaces. Indeed, in our study, side effects were mild and did not cause interruption of CPAP treatment in any patient.
Median total unintentional and intentional leaks were measured as 68 l/min (IQR 63–75). In a preliminary bench study evaluating this configuration,18 the amount of only intentional leaks was 43.6 l/min at 10 cmH2O and 48.6 l/min at 12 cmH2O using a ID 5.5 mm connector. We can speculate that the use of a larger I.D (6 mm) could have led to this result. In six patients (17.1%), more than two replacements of the interface every three hours were needed, due to a “positive leak test” (>10 l/min). Real-time leaks monitoring allowed quick identification of helmet displacements and subsequent repositioning.
Although suggested by other authors,31 active humidification was not used in this study and HEPA filters were positioned on the two helmet ports, limiting the risk of infection related to condensation removal procedures. Mucus plugging never occurred. As turbine-driven ventilators are fed by room air instead of high-pressure dry air, we can speculate that a better natural airway humidification was obtained in the proposed configuration.
The ATS / ERS guidelines in 2017 stressed that every trial on the use of NIV must be managed by a team of experts and patients should be closely monitored, in order to avoid any delay in intubation, also in consideration of the increased risk of SILI.3,32 Surviving Sepsis Campaign did also not recommend CPAP treatment for the initial management of COVID-19.33 However, a European consensus document suggested using h-CPAP in COVID-19 mainly with the aim of reducing aerosol generation 4,5,34 and other studies successfully used h-CPAP in the COVID-19 pandemics.35–39 The failure rate of 34.3% (described as the rate of intubation and/or death) described in our study, is comparable with the ones observed in similar settings by Franco et al.36 and Aliberti et al.39 (47.3% and 44.6%, respectively). The overall mortality rate of 28.6% appears to be consistent with data coming from two large Italian studies (ICU setting, mortality rate 26%40; pneumology units, mortality rate 30.3%36) with similar populations (median PaO2:FiO2 ratio 16040; median PaO2/FiO2 ratio 15136) if compared to our population (PaO2:FiO2 ratio median 129).
Our study has several limitations. Firstly, the study did not include a control group and we did not compare our ventilator configuration with others. However, to the best of our knowledge, there are no other commercially available TDVs configurations able to estimate Vt in a reliable way. Secondly, although different tools may be used to assess Vt non-invasively during h-CPAP, these are not clinically applicable on a long term basis.41 Finally, our study design was intended as a preliminary evaluation of the effectiveness and safety of the new h-CPAP configuration in a clinical setting. Thus, speculations on the overall efficacy of h-CPAP in COVID-19 was outside its scope.
ConclusionsThe use of h-CPAP for treating COVID-19 related ARF using high-performance turbine-driven ventilators in a single-limb intentional leak configuration allowing Vt monitoring may be reliable, effective, and safe for both patients and healthcare workers. Further studies evaluating this configuration in larger patients’ cohorts are needed.
FundingNone.
Ethics approvalCORACLE Registry – “Città della Salute e della Scienza di Torino” University Hospital, ID number 0031285, date 24 March 2020.
Consent to participateAcquired from all participants.
Authors' contributionsCC and AM conceived the content, retrieved the data, wrote the manuscript and approved the final version. GM, MI, AC gave important intellectual contributes to data interpretation, wrote the manuscript and approved the final version. CA, EP revised the manuscript for important intellectual content and approved the final version. CG conceived the content, wrote the manuscript and approved the final version.
The authors acknowledge William Truschel, B.S., (Former Principal Scientist, Philips Respironics Sleep and Respiratory, Murrysville, Pennsylvania) for providing technical and intellectual help in this project.